Lípidos: Composición, estructura y función
por Nathan H Lents, Ph.D., Lizzie Stark, M.S./M.F.A, Bonnie Denmark, M.A./M.S.
¿Sabías que estudiar los lípidos puede ayudarnos a comprender y tratar afecciones médicas como enfermedades cardíacas, trastornos hormonales, esclerosis múltiple y muchas otras? Los lípidos son necesarios para la estructura de todas las células vivas. Su composición química les permite tener muchas funciones importantes, desde almacenar energía hasta regular el metabolismo y ayudar al pelaje de las nutrias marinas a repeler el agua.
Lipids are a large and diverse class of biological molecules marked by their being hydrophobic, or unable to dissolve in water.
The hydrophobic nature of lipids stems from the many nonpolar covalent bonds. Water, on the other hand, has polar covalent bonds and mixes well only with other polar or charged compounds.
Fats and oils are high-energy molecules used by organisms to store and transfer chemical energy. The distinct structures of different fat molecules gives them different properties.
Phospholipids are specialized lipids that are partially soluble in water. This dual nature allows them to form structures called membranes which surround all living cells.
- enlace
- el acto de unir dos átomos
- membrana
- capa de tejido que forma el límite de una célula
- polar
- llevar una carga eléctrica
What do butter, beeswax, and testosterone have in common? They’re all lipids, a type of compound produced by plants and animals that includes fats and oils as well as waxes and steroids. As a group, lipids have many different functions and uses in living cells and organisms, from storing energy to regulating metabolism, signaling hormones, and providing the structure of cell membranes. They help sea otters’ fur repel water and give a waxy sheen to many plant leaves. In our daily lives, lipids provide the delicious richness in ice cream, give carrots their color, lubricate our car engines, and help clean our clothes.
What is a lipid?
If you have ever made salad dressing, seen a photograph of an oil tanker spill, or tried to clean a greasy stain with water, then you have likely noticed one of the defining factors of lipids: They do not mix well with water. Lipids are mainly composed of carbon and hydrogen atoms, and this hydrophobic ("water fearing") nature of lipids is driven by the bonds between these many carbons and hydrogens.
In a water molecule, the bonding between the oxygen and hydrogen atoms results in a polar covalent bond (see our module Water: Properties and Behavior). The electrons that form this bond are shared unequally between the atoms because oxygen atoms have a stronger pull on electrons than hydrogen does. This creates a slight negative charge at the oxygen end of the water molecule, and a slight positive charge at the hydrogen end, as shown in Figure 1.
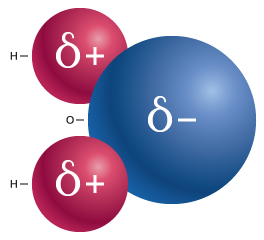
However, the bonding between carbon and hydrogen atoms in lipids is not polar. This is because the electrons in the covalent bonds are shared equally between the carbons and the hydrogens and there are no partial charges anywhere. Thus, long chains of carbon-hydrogens bonds form a nonpolar molecule.
The bonding differences between water and lipid molecules is important because “like attracts like.” As a polar solvent, water prefers to dissolve molecules with polar bonds, such as salt and sugar. Molecules with nonpolar bonds will not normally dissolve in polar solvents because there is no charge on the nonpolar molecule to attract the polar molecule. Nonpolar liquids mix with other nonpolar liquids and dissolve nonpolar solutes (the substance that is dissolved); polar liquids mix with other polar liquids and dissolve polar or charged solutes.
While lipids cannot dissolve in polar solvents, they can dissolve in nonpolar solvents – those with a balanced electron distribution – such as gasoline and chloroform. This is why lighter fluid can help remove engine grease and cooking oil stains from clothing.
Punto de Comprensión
Lipid compounds
As a group, lipids are a diverse collection of naturally-occurring organic compounds with important roles to play:
- Fats and oils store energy for cells. In animals, they provide electrical insulation for nerves, and cushion internal organs.
- Phospholipids form cellular membranes and play an important role in diffusion (see our Membranes I: Introduction to Biological Membranes module).
- Steroids are formed from cholesterol and are involved in cellular communication.
- Carotenoids are pigments used to help absorb light energy in plants, algae, and photosynthetic bacteria.
- Waxes form a barrier to exclude water in both plants and animals. Waxes are found in leaves, ear canals, and the beeswax that makes honeycomb.
The composition of lipids
Without fully realizing it, humans have been performing chemical reactions with lipids for thousands of years. Soap, for example, was a very early human invention and possibly the first such innovation to be the result of a chemical reaction. There is even a recipe for making soap on Sumerian tablets dating back to 2500 BCE (Levey, 1954). In the ancient world, soap was made by first boiling rainwater with ashes from burnt wood to produce lye: a very basic, or alkaline, solution (high pH) (see our Acids and Bases: An Introduction module). Next, this solution was combined with animal fat or vegetable oil and cooked over a low fire for many hours until the mixture changed into a gel. The fundamental procedure of this chemical reaction, now called saponification, is still used today to make soap.
The first steps toward understanding lipids were taken in the early 1800s by a young French scientist named Michel Chevreul (1786-1889). Chevreul began his career in the laboratory of Louis Vauquelin, where his role was to use various solvents (such as water, alcohol, and ether) to separate the colored dye pigments from natural products like vegetable oils, waxes, tree gums, and resins. Without knowing it, he was working with various kinds of lipids (McNamara, Warnick, & Cooper, 2006).
At the end of each experiment, Chevreul would wash out the glassware using a lot of soap. While conducting his research, Chevreul observed that if he accidentally left soapy water in some glassware and it evaporated overnight, salt crystals would be left behind. He was confused by this because he had added only water (or another solvent) and soap to the glassware. It raised the question: Where was the salt coming from? Through deductive reasoning, Chevreul realized it must be the result of the soap. When he learned how soap was made by mixing animal or vegetable fat with alkali water, though, he was still confused because there was no salt in that process either.
Intrigued and persistent, Chevreul went on to study the process of soap-making in his own laboratory. As he made various kinds of soap, he observed that as oils react with the alkali water, they turn from a translucent liquid into a thick, milky pudding, which gradually hardens. At the time, he knew that oils and fats contain large amounts of carbon and hydrogen and only small amounts of oxygen. He hypothesized that the reaction with the alkali solution, which had a high pH and thus a higher concentration of hydroxide ions (OH-), was somehow adding oxygen atoms to the structure of the fats to change them from pure hydrocarbons to molecules with some salt-like properties.
This was an excellent hypothesis because it would explain two different phenomena at the same time. First, it explained the salt crystals left when soapy water dries. Second, it explained why soap is soluble in both water and oil. The hydrocarbons from the fat would still be oil-soluble, but their new salt-like properties, coming from the added oxygen atoms, would allow them to be soluble in water, a property that all salts have.
Punto de Comprensión
The structure of fats
Although it took him most of his career to do it, Chevreul demonstrated that his hypothesis was correct. He did this by performing painstaking chemical analyses of various fats, oils, and the soaps that are produced when alkali is added to them. Chevreul discovered that, during saponification, some of the hydroxide (OH-) ions from the alkali solution are indeed added to the hydrocarbons from the fats. When this happens, some chemical bonds in the fat molecules are broken, releasing long-tailed fatty acids (Figure 2). Many of the names of common fatty acids that we use today were given to these molecules by Chevreul (Cistola et al., 1986).
Figure 2: The basic chemical reaction of saponification.
The reason that hydrocarbon tails from fats are not soluble in water is because almost all of the bonds are symmetrical and thus nonpolar. However, when the hydroxide ions break the ester group in fat molecules during saponification, a charged and polar group is created – a carboxylic acid group – which is very soluble in water.
These fatty acids have a very special structure. They have long chains of nonpolar bonds, which makes them easily dissolvable in oil and grease; but they also have a polar charged group at one end, which makes them easily dissolvable in water. Thus, these molecules have a dual nature – they are both water-soluble (hydrophilic, "loves water") and oil-soluble (lipophilic, "loves fat"). The word for this is amphiphilic, which means "loves both." This is why fatty acids perform so well as soaps and detergents – they are capable of dissolving, and thus cleaning, both watery and greasy substances.
What Chevreul and others showed was that an alkali solution breaks up the fat molecules and two parts are released: glycerol and fatty acids. We now know the complete structure of the fat molecule (Figure 3).
Figure 3: A fat molecule showing its component parts: the glycerol, carboxyl groups, and fatty acids. From Harrigan, G.G., Maguire, G., and Boros, L. 2008. Metabolomics in alcohol research and drug development. Alcohol research Health, 31(1): 27-35.
image ©Harrigan, G., Maguire, G., and Boros, L.During the process of saponification, the hydroxide ions in the alkali solution "attack" the ester group and thus release the fatty acid chains from the glycerol backbone. Chevreul was able to figure this out by analyzing the chemical composition of the fats before the reaction, and then repeating the analysis with the fatty acids that resulted. He did this again and again with different kinds of fats, which made slightly different kinds of soaps. The result was the common theme that fats are made of glycerol and fatty acids.
Fats and oils store energy
Animals and plants use fats and oils to store energy. As a general rule, fats come from animals and oils come from plants. Because of slight differences in structure, fats are solid at room temperature and oils are liquid at room temperature. However, both fats and oils are called triglycerides because they have three fatty acid chains attached to a glycerol molecule, as shown in Figure 3.
The carbon-hydrogen bonds (abbreviated C-H) found in the long tails of fatty acids are high-energy bonds. Thus, triglycerides make excellent storage forms of energy because they pack many high-energy C-H bonds into a compact structure of three tightly packed fatty acid tails. For this reason, dietary fats and oils are considered "calorie dense." When animals, including humans, consume fats and oils, a relatively small volume can deliver a large number of calories. Animals, particularly carnivores, are drawn to high-fat foods for their high caloric content.
Triglycerides are formed inside plant and animal cells by attaching fatty acids to glycerol molecules, creating an ester linkage. This reaction is called a dehydration synthesis because a water molecule is formed by "pulling out" two hydrogen atoms and an oxygen from the reactants. Because a new water molecule is formed, this new reaction is also called a condensation reaction (see Figure 4).
Figure 4: The dehydration synthesis reaction, where a water molecule is formed by "pulling out" two hydrogen atoms and one oxygen atom.
Punto de Comprensión
Structure of fatty acids
The reason why fats are solid at room temperature while oils are liquid has to do with the shape of the fatty acids these triglycerides contain. Remember that the fatty acids are long chains of carbon molecules that have hydrogen atoms attached. The C-H bonds are where energy is stored. At one end of the tail, fatty acids have a carboxyl group (-COOH), which gives the molecule its acidic properties (Figure 5).
Figure 5: The essential features of a fatty acid showing the long hydrocarbon chain and the carboxylic acid group.
If a fatty acid looks like the molecule above, with only single bonds between the carbons, we say that this fatty acid is saturated. This term is used because every single carbon is surrounded by as many hydrogen atoms as is possible; it is saturated with hydrogen.
However, some fatty acids have a double bond between two of the carbons in the chain. Wherever this double bond exists, abbreviated C=C, both of the carbons involved in this double bond have one less hydrogen than the other carbons. This is because carbon can only normally make four bonds. When two carbons form a second bond in between them, they each must "let go" of a hydrogen so that the total number of bonds for each carbon is still four. Because these fatty acids have two fewer hydrogen atoms than they otherwise would have, we call them unsaturated fatty acids (Figure 6). They are unsaturated because they do not contain the maximum number of hydrogen atoms that they could have.
Figure 6: A mono-unsaturated fatty acid.
When a fatty acid has a double bond in its chain, the chain has a "kink" in its shape because there is no free-rotation around a C=C double bond. The kink is "fixed" in the structure of the fatty acid. In contrast, saturated fatty acids have free rotation around all of the single bonds in the chain since saturated fatty acids are long and straight. A comparison is shown in Figure 7.
The kinks found in unsaturated fatty acids make it so that many chains cannot pack together very tightly. Instead, the kinks force the fatty acids to push further apart. For this reason, triglycerides with unsaturated fatty acids are liquid at room temperature. Instead of packing together tightly, the molecules can slide past each other easily. The opposite is true for triglycerides with saturated fatty acids. Because their fatty acid tails are straight with no kinks, they can pack together very tightly. Thus, these molecules are more dense and solid at room temperature.
Figure 7: A comparison of a saturated fatty acid (stearic acid, found in butter) and an unsaturated fatty acid (linoleic acid, found in vegetable oil).
Animal fats are often saturated, which explains why lard, bacon fat, and butter are all solid at room temperature. Plant triglycerides, on the other hand, are typically unsaturated. This is why vegetable oils (such as canola, olive, peanut, etc.) are liquid at room temperature. Most often, unsaturated fats have only one C=C double bond and are thus called monounsaturated. However, some plants make triglycerides with multiple C=C bonds. These kinds of triglycerides are called polyunsaturated. (See Figure 8.)
Figure 8: A comparison of the bonds in a monounsaturated fatty acid (oleic acid) and a polyunsaturated fatty acid (linoleic acid).
Monounsaturated fats appear to be the healthiest triglycerides for humans to consume in their diets because the cells that remove fats from our blood after they are absorbed from our diet do their work most quickly with monounsaturated fats. Because we are slower to remove them from our blood, saturated fats stay in our bloodstream longer and thus have a greater chance to contributing to the formation of plaques and clots. For this reason, doctors and dieticians recommend diets high in monounsaturated fats and low in saturated fats. Polyunsaturated fats are somewhere in between saturated and monounsaturated fats in terms of their healthiness in our diet (Mattson & Grundy, 1985).
Punto de Comprensión
Trans fats
Another type of fatty acid that has gotten a lot of attention recently is the trans fatty acid. Trans fatty acids have a hydrocarbon tail with a double bond that is in the trans configuration, instead of the more common cis configuration (see Figure 9).
Figure 9: A comparison of the cis double-bond configuration and the trans double-bond configuration.
As discussed above, C=C double bonds are present in the fatty acid tails of unsaturated fats. When these unsaturated fatty acids are made naturally by living cells, most often plant cells, the C=C double bonds are always in the cis configuration, almost never in the trans configuration. However, during industrial production of certain fat-containing products, the trans configuration can be inadvertently formed. This occurs when unsaturated fats, usually vegetable oils, are subjected to the process of hydrogenation in order to turn them into saturated fats (shown in Figure 10).
Figure 10: Unsaturated fats, usually vegetable oils, are subjected to the process of hydrogenation in order to turn them into saturated fats.
The purpose of industrial hydrogenation is to create solid fats, which are more desirable for deep-frying, out of vegetable oils. This is done because vegetable oils are much less expensive than naturally saturated fats such as lard. Crisco™ and margarine are two such chemically-produced saturated fats that are made of hydrogenated vegetable oils. Crisco™, or shortening, is cheaper than lard but can be used similarly and gives similar taste. Margarine, or oleo, was developed as a cheaper substitute for butter, particularly during the era of the World Wars and global depressions that marked the first half of the 20th century, when rationing and scarcity of staples was common. Today, many packaged desserts and candies also have these kinds of industrially produced saturated fats, which often cost less than natural saturated fats but provide better texture and firmness than unsaturated fats. During hydrogenation, occasionally the chemical reaction does not go to completion and the process of turning a cis unsaturated fat into a saturated fat creates a trans fat instead.
In recent years, trans fats have received a lot of attention from dieticians and the general public because of their association with elevated health risks. Individuals with diets higher in trans fats are more likely to develop coronary heart disease, suffer heart attacks and stroke, and die earlier than those with diets low in trans fats (Mensink & Katan, 1990). It was always known that hydrogenation produces some trans fats, but because they are not acutely toxic, their long-term health dangers are only now being realized.
Scientists have discovered the reason for these elevated risks: Trans fats spend a much longer amount of time in our bloodstream after we consume them, instead of being quickly absorbed into our cells. Unlike saturated fats and cis unsaturated fats, trans fats don't appear in nature in very large amounts – they are an "unnatural" form of fat which humans are not well designed to consume. Because humans only began to eat trans fats in the 20th century (other than the very tiny amounts that are present in some forms of red meat), we do not have receptor molecules in our blood vessels that seek out these trans fats and remove them from the bloodstream. Thus, when we consume trans fats, they persist in our bloodstream for a very long time, compared to natural forms of fat. The longer these molecules spend in our bloodstream, the more they can contribute to the formation of clots, plaques, and hardened arteries. For this reason, the United States Food and Drug Administration has recently made a preliminary determination that trans fats are “not generally recognized as safe,” a determination that will likely lead to a complete ban on their presence in foodstuffs (Brownell & Pomeranz, 2014).
Punto de Comprensión
Phospholipids and glycolipids form cellular membranes
Perhaps the most important and basic function of lipids in living cells is in the formation of cellular membranes. All cells, from the most basic bacterium to those that form the most specialized human tissues, are surrounded by a plasma membrane made of lipid molecules. For more detail, see the Membranes I: Introduction to Biological Membranes module.
The lipids that form membranes are a special type called phospholipids (Figure 11). They are so named because they have a characteristic phosphate group (PO4). Like triglycerides, the central structure of a phospholipid is the glycerol molecule. However, phospholipids have two fatty acid tails attached to the glycerol, whereas triglycerides have three. On the remaining carbon of the glycerol, a large, charged, phosphate-containing group is added.
Figure 11: A phospholipid.
image ©OpenStax CollegeThis distinctive head group gives phospholipids their unique properties. Like fatty acids, the presence of a hydrophobic tail and a hydrophilic head means that phospholipids are amphiphilic. This distinctive structure leads to a very peculiar behavior by phospholipids – the spontaneous formation of bilayers. When phospholipid molecules are placed into an aqueous solution (water-based), they will arrange themselves into sphere-shaped structures in which the surface of the sphere is a double layer of phospholipids. While the hydrophilic head groups are attracted to the water in the surrounding solution, the hydrophobic tails are repelled by it and attracted to each other. This means that the most “comfortable” arrangement for the phospholipids to take is to tuck their tails together in a water-free interior space, with the polar head groups facing out, interacting with water (Figure 12) – this is called a micelle<. You can learn more about the ways in which phospholipids function in the Membranes I: Introduction to Biological Membranes module.
Figure 12: Three of the different structures phospholipids can form in an aqueous solution: micelle, liposome, and bilayer sheet. In this depiction, the hydrophilic heads are round and white and the hydrophobic tails are yellow wavy lines.
Punto de Comprensión
Steroids provide structure and cell signaling
Another class of lipid molecules that are important in cells are the steroids, also called sterols. Unlike triglycerides and phospholipids with their long hydrocarbon tails, steroids consist of four fused carbon rings, as shown in Figure 13. As you would expect because of all of the nonpolar C-H bonds, steroids are not soluble in water.
Figure 13: The generic structure of a steroid molecule and the structure of cholesterol.
Cholesterol
The most fundamental steroid molecule is cholesterol because all of the other steroids that are made from it. Cholesterol has its own functions as well. For example, in animal cells, cholesterol is embedded in cell membranes to give them fluidity and to prevent them from solidifying in cold temperatures. Plants contain molecules similar to cholesterol called phytosterols that perform similar functions.
Cholesterol was named by Michel Chevreul in 1815, who found that human gallstones have a large amount of this lipid. A century later, Alfred Windaus and Henrich Wieland confirmed that the liver made cholesterol, although they deduced its structure incorrectly. They shared the Nobel Prize in 1928 for their discovery that cholesterol and other bile acids are made by the liver and used to dissolve dietary fats so that they can be absorbed by the intestines. The correct structure of cholesterol wasn't confirmed until 1945, when Dorothy Crowfoot Hodgkin used the new technique of X-ray diffraction (see Figure 14) to realize the precise arrangement of the four-ring structure (Bloch, 1982).
Figure 14: An x-ray diffraction pattern.
image ©Jeff DahlOther steroids
There are many other steroids, but all of them, by definition, are cholesterol derivatives (Figure 15). That is, they are made using cholesterol as the starting material. Many of these steroids are hormones, such as the sex steroids estrogen, progesterone, testosterone, and their cousins. Other steroid hormones include cortisol and aldosterone.
Figure 15: A chart of the steroid hormones and their biosynthetic relationships.
image ©David Richfield and Mikael HäggströmAlthough these hormones all perform widely differing functions in the body, they have a strikingly similar structure. This common structure means that they have a similar mechanism of action. Steroid hormones are released by glands and then travel throughout the body where they exert their actions by binding to their receptors inside of cells and then activating or de-activating genes. The power of steroid hormones is in their lipid nature, which allows them to cross biological membranes easily. Thus, a hormone produced in one tissue will quickly and easily diffuse throughout the entire body, passing through cells as easily as oxygen and carbon dioxide do (see Figure 16.)
Figure 16: A steroid hormone receptor's mechanism of action.
image ©DesignuaOther lipids
Several other sorts of compounds are grouped in with the lipid family because they are insoluble in water.
Carotenoids
The pigments that give some plants their orange and yellow color (e.g., carrots and summer squash) are carotenoids. They contain branching five-carbon chains called isoprene units (see Figure 17). Animals are able to break down these molecules into vitamin A, which may then be used to produce retinal, a pigment necessary for eyesight.
Figure 17: Isoprene units contain branching five-carbon chains. Animals are able to break down these molecules into vitamin A.
Waxes
Waxes appear in many different living things, providing the natural coating on some leaves and fruits, the sheen on the feathers of some birds, the shine on human hair, and the protective secretions in our ear canals. Like triglycerides, waxes are esters of fatty acids, consisting of an alcohol molecule bonded to fatty acids through ester linkage. Wax is strongly hydrophobic, and thus serves as an effective water repellant. In addition, the fully saturated hydrocarbon chains of wax molecules makes them solid at room temperature, like saturated fats discussed earlier (see Figure 18).
Figure 18: A wax molecule showing the long-chain alcohol and fatty acid.
Punto de Comprensión
Lipid research and medical science
Lipids play a role in eyesight, nerve tissue, vitamin absorption, the endocrine system, and many other body functions. Scientists have known that some fat is carried in the bloodstream ever since the late 1600s, when researchers examined the blood of animals that had just eaten a fatty meal and discovered that it briefly turned milky and yellowish. Now it’s clear that an excess of cholesterol in the blood can lead to deposits called plaque in artery walls, which increases a person’s risk of heart attack. Research into these fatty plaques has revealed that trans fats strongly exacerbate their formation, given how much longer they persist in the bloodstream. In addition, chemicals from cigarette smoke have been shown to increase the inflammatory response that gradually turns these fatty deposits into plaques and then to obstructive clots. Fortunately, arterial plaques are dynamic, and their formation can be reversed by stopping smoking and transitioning to a diet lower in cholesterol and fats from the saturated and trans fats family.
Ongoing research in lipid chemistry advances medical knowledge as we seek to understand and treat high cholesterol, heart disease, hormone disorders, thyroid disease, fatty liver disease, multiple sclerosis, autism spectrum disorder, macular degeneration, Guillain-Barré syndrome, and other conditions.
Tabla de Contenido
Active el resaltado de términos del glosario para identificar fácilmente los términos clave dentro del módulo. Una vez resaltados, puede hacer clic en estos términos para ver sus definiciones.
Active las anotaciones NGSS para identificar fácilmente los estándares NGSS dentro del módulo. Una vez resaltados, puede hacer clic en ellos para ver estos estándares.