Acids and Bases II: Conjugate ions and buffers
by Robin Marks, M.A.
We think of water as neutral, but did you know that water can act as both an acid and a base? This is one of water’s most important roles in our body chemistry. Key to the body’s pH-control system is this property of water that allows it to donate or accept protons to keep our body fluids and tissues in a state of equilibrium. Acid-base chemistry is also important to many other common processes such as dying fabrics, producing everyday grooming products, and manufacturing life-saving medications.
The Brønsted-Lowry system defines a conjugate base as an acid without its proton, and a conjugate acid as a base with an additional proton.
Water is amphoteric, meaning it can act as both an acid and a base, accepting a proton to become a hydronium ion (H3O+), or dissociating into a proton and a hydroxide ion (OH-).
The strength of an acid is based on its tendency to ionize in water, not on the concentration of the acid in a solution
Buffers are solutions containing a weak acid and its conjugate base, allowing them to absorb a strong acid or base without much change in pH.
- acidosis
- a condition in which your body’s pH is out of balance and the blood and body tissues are too acidic.
- hydronium ion
- also called hydronium, this is the positively charged molecule that results in an acidic solution when water (H2O) acts like a base and accepts a proton (H+), becoming H3O+.
Ah, pizza—and the indigestion it can cause. In our Acids and Bases I: Definitions, pH and neutralizationI module, we looked how antacids can act as a base to neutralize pizza’s acidic tomato sauce and cheese. Antacids contain calcium carbonate, which dissociates and releases a carbonate ion (CO3
But even if you don’t take an antacid, your body manages to adjust your digestive system to the proper pH. And your body is constantly producing carbon dioxide, some of which you breathe out, but some of which stays in your blood and combines with water to create carbonic acid. So how does your body maintain a pH that keeps it functioning, regardless of what you’re exposed to?
Physiologists and chemists became very interested in this question of internal acid/base balance in the late 1800s, driven by a desire to treat people for whom that balancing mechanism wasn’t working, a condition called acidosis (a condition in which acid builds up in the blood). These scientists’ efforts to better understand how the body achieves its pH balance resulted in a much richer picture of what actually happens between acids and bases in solution. This knowledge continues to play an important role in treating acidosis, which is common among people with kidney disease or diabetes.
Equilibrium: A Balancing Act
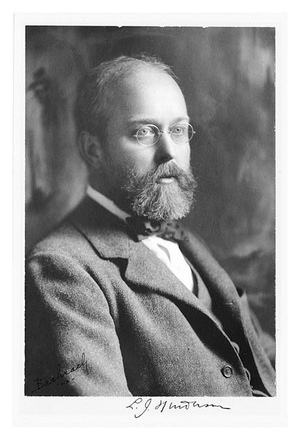
In the early 20th century chemists began to clearly understand how ions, including those of acids and bases, behave in water. A promising young Harvard biochemist named Lawrence Henderson applied this new understanding to biological systems. He knew that patients with acidosis improved when they got an injection of bicarbonate, which occurs naturally in the body. Henderson studied the equilibrium and dissociation reactions of bicarbonate, and, in a landmark paper, he described his conclusion that these ions and other weak acids made up the body’s pH-control system.
What went into Hendersonâs reasoning? He knew that carbonic acid dissociates in water, releasing a proton:
Research at the time was revealing that this equation is only partially correct, because it leaves water out of the picture. But water actually plays an important role in acid-base chemistry. In an acidic solution, water acts like a Bronsted-Lowry base by accepting a proton released by the acid (See our Acids and Bases I: Definitions, pH and Neutralization module to review this definition of acids and bases):
The resulting positively charged molecule is called a hydronium ion (H3O+). In fact, this reaction to form hydronium ions even occurs in a glass of pure water. Because water is polar, and is constantly engaging in hydrogen bonding with other water molecules, a very small number of those molecules dissociate into their constituent ions as in equation 4 below:
The two-sided arrow shows that the reaction happens in both the forward direction (left to right) and the reverse direction (right to left) at the same time. In other words, while some water molecules are taking on a hydrogen ion to become hydronium and forming hydroxide (OH-), some hydronium ions are losing a hydrogen ion to become water. When the forward reaction is happening at the same rate as the reverse reaction, a condition we call equilibrium, the pH of water is 7.0, which is what we consider neutral.
However, this reaction can be influenced by other components in the water – add a base to water and some of the hydronium ions react without to form water. Add an acid to water, and you shift the equilibrium towards formation of more hydronium ions. Water is, in fact, one of several molecules with the capacity to act both as an acid and as a base. Such molecules are called amphoteric.
Adding the role that water plays into Equation 2, we get a more complete picture of whatâs happening in the carbonic acid solution:
Thus, water isn’t simply a bystander, but rather participates in the reaction. The addition of acid to the solution shifts the equilibrium towards the creation of hydronium ions (H3O+), making the solution acidic. This is true for all acid-base reactions.
Comprehension Checkpoint
Conjugate Acids, Bases & Amphoteric Molecules
Let’s look a little more closely at how this plays out with our carbonic acid example. Equation 5 shows that water has accepted a dissociated proton from the carbonic acid. As we saw, in water, carbonic acid dissociates according to the equation:
In this reaction (Eqn. 5), carbonic acid donates a proton and water acts as a base by accepting the proton. The resulting products are a bicarbonate ion that formed when carbonic acid lost its proton and the hydronium ion that formed when water accepted it. The hydronium ion is called the conjugate acid of water. A conjugate acid of a reaction is the species that has accepted the proton from the reactant that donated it. In this case, the hydronium ion is able to react as an acid in another reaction, donating the proton it gained. The bicarbonate ion is the conjugate base, the species that lost a proton in the initial reaction, A conjugate base is able to accept a proton in another chemical reaction.
This phenomenon always happens in pairs. The acid in a reaction always produces a conjugate base when it gives up its proton, and the original base always produces the conjugate acid when it accepts the proton. The four pieces always exist together, whether it is water acting on the original acid or another compound.
The two-sided arrow in Eqn. 5 tells us that the reaction is happening in both the forward and reverse directions. In a solution of carbonic acid and water, acid and base molecules are constantly reacting to form conjugate base and conjugate acid molecules, and at the same time, the conjugate acid and base molecules are reacting to form the original acid and base
A similar phenomenon happens when a base is mixed with water. Remember that water is amphoteric, able to act as both an acid and a base, depending on the conditions. Let’s look at what happens when we put a base, in this case ammonia (NH3), in water:
In the forward direction of this reaction, water is donating a proton to NH3. In the reverse direction, the OH- ion acts as the conjugate base, because it can accept a proton donated by the conjugate acid, NH4+.
Equation 3 and Equation 4 demonstrate how water is amphoteric. It turns out that the bicarbonate ion Lawrence Henderson wrote about in the early 1900s is also amphoteric and can act as either an acid or a base. In other words, bicarbonate can accept a proton, as the conjugate base does in the reverse reaction in Equation 5, or it can donate a proton, to become a carbonate ion (creating a hydronium ion in the process).
While Henderson had uncovered a central clue in the story of how the body maintains its pH balance, he wasn’t able to explain exactly how that happens until he read the work of another chemist.
Comprehension Checkpoint
Strong versus weak acids
Before getting further into our explanation of how bicarbonate controls blood pH, we need to define some differences between strong and weak acids. Hydrochloric acid, HCl, is a strong acid that’s found in your stomach, where it helps break down food. It can also break down fabric or skin, leaving burn holes in both. Carbonic acid, on the other hand, is a weak acid. Not only can you spill it all over your clothes and skin without consequence, you can pump it through your entire body.
So, what makes one acid strong and another weak? It is not the amount of acid that is used but rather the amount of acid that actually ionizesâdissociates into its constituent ionsâin water.
When you add hydrochloric acid to water:
All of the HCl molecules dissociate, leaving only H+ and Cl- ions, and there are no molecules of HCl remaining. This reaction only goes in the forward reaction (thus the single-headed arrow in the equation); the resulting products remain dissociated. Acids that completely dissociate in water are referred to as strong acids.
Dissolving carbonic acid in water, however, is different:
While some of the carbonic acid dissociates into ions, some of those resulting ions will join together to form carbonic acid again. Thus, at any given time, there will be both carbonic acid molecules and bicarbonate ions in the solution. This is the definition of a weak acid, one that does not dissociate completely in water. In solutions containing weak acids, both the forward and reverse reactions are always happening at the same time, and we indicate this by using the two-sided arrow in the equation.
These definitions of strong vs. weak apply to bases as well. NaOH (sodium hydroxide), a strong base, completely dissociates in water:
This leaves only ions, and no NaOH molecules in the solution. Ammonia (NH3), on the other hand, is a weak base:
At any given moment, some ammonia molecules will remain uncharged while others take on a proton from water to become ions.
Weak acids and their conjugate bases make good buffers
At about the same time that Henderson was studying the bicarbonate system, the Danish chemist Sören Sörenson was investigating a concept that would explain how bicarbonate works its magic. Sörenson was interested in biological molecules called enzymes, protein molecules that catalyze chemical reactions in living things. Sörenson noticed that enzymes work best within a very small pH range. His study of how proteins maintain this range led to the paper in which he proposed his now-famous pH scale.
That paper was published just a few months after Henderson’s study of bicarbonates. Along with describing the pH scale, Sörenson’s paper explained some experiments he had done to discern how the body maintains the narrow pH range an enzyme requires. If he added a weak acid to water, the pH would go down. No surprise there. But, Sörenson discovered, if he added that same weak acid and its sodium salt (the weak acid combined with a sodium ion), the pH barely budged. In this way, he effectively added more conjugate base to the solution, and found that it stabilized the hydrogen ion concentration. We can explain why by looking at the ions involved.
We already know that carbonic acid dissociates according to this equation (Eqn. 5):
Sodium bicarbonate (which is the chemical name for baking soda), dissociates in this way:
Notice that the sodium bicarbonate reaction only goes in the forward direction and doesn’t involve hydronium ions because it isn’t actually an acid-base reaction, just the dissolving of a solid. The net effect of adding the sodium bicarbonate is really adding bicarbonate ions, in other words, the conjugate base of carbonic acid.
If carbonic acid (or any other acid) is added to this solution,the extra bicarbonate ions are available to absorb the acidic protons, minimizing changes in pH. Without those extra bicarbonate ions, the H+ from the additional carbonic acid would be released into solution until the system reached equilibrium, with a higher [H+]. Instead, the bicarbonate added by the baking soda absorbed those ions, forming more carbonic acid while maintaining a stable pH.
Sörenson described this process as “buffering,” and called the solution of weak acid plus conjugate base a buffer. He likened it to adding shock absorbers on a train. The springs in the shock absorbers absorb the train’s up and down bounces, giving passengers a smoother ride. Similarly, the conjugate base of a weak acid acts as a “proton absorber, able to absorb the addition of new protons and keep the pH within a certain range. Buffers using a weak base and its conjugate acid work in the same way.
When Henderson read Sörensonâs description of this concept, he had his explanation of how the bicarbonate system maintains blood pH: bicarbonate ions in the blood are able to absorb excess protons. In fact, there are bicarbonate ions in all the fluids in your body. At the beginning of this module, you learned that your body is always producing carbon dioxide as a waste product of metabolism, and some of it combines with water to create carbonic acid. That carbonic acid dissociates when it combines with water in your cells and body fluids. The equilibrium works like this:
Your kidneys regulate the concentration of bicarbonate in your blood, making sure there are always enough of these ions. This role of the kidneys explains why people with kidney disease and diabetes are susceptible to acidosis. If the kidneys aren’t functioning properly, they fail to retain bicarbonate ions, and so the body loses some of its buffering capacity.
Comprehension Checkpoint
Conclusion
The bicarbonate buffer system offers an elegant example of the potential of acid-base chemistry. Strong acids and bases, which completely dissociate in water, can induce burns and corrode metal. Weak acids, however, can produce a wide range of pH’s, and are important in any system that requires a pH closer to the middle of the spectrum. The combination of a weak acid with its conjugate base can create a buffer to mitigate the effects of additional protons from other acids or reactions in the solution. This buffering capability is central to the success of any process that requires maintaining a steady pH, such as dying fabrics, manufacturing drugs, counteracting acidity in shampoo and baby lotion, and even keeping your pizza from giving you acidosis.
Table of Contents
Activate glossary term highlighting to easily identify key terms within the module. Once highlighted, you can click on these terms to view their definitions.
Activate NGSS annotations to easily identify NGSS standards within the module. Once highlighted, you can click on them to view these standards.